MOOC: Instrumental analysis of cultural heritage objects
4.2. XRF and XRD
In this lecture, two methods – X-ray fluorescence spectroscopy (XRF) and X-ray diffraction (XRD) analysis – will be discussed together. During the lecture, sample preparation will be described, and practical tips for the analysis as well as examples will be given. XRF (more recently also portable XRF) is (together with SEM-EDS) among the most important methods for determining the elemental composition of cultural heritage materials. XRD is valuable for analysing the structure of crystalline materials, i.e. the spatial arrangement of atoms in crystals and identifying the materials on the basis of the structure. XRD is applicable to minerals, clays etc.
1. General aspects of X-Ray Diffraction and X-Ray Fluorescence
In the following video, the general theoretical aspects of XRD and XRF are discussed.
Description of X-ray fluorescence spectroscopy (XRF)
All X-ray fluorescence spectrometers share the common principle that can be presented as follows:
- Polychromatic (i.e. containing photons with different energies/wavelengths) X-rays are directed onto the sample. Polychromaticity of the radiation is needed in order to enable, as far as possible, detecting the atoms of all the different elements that are present in the sample.
- The X-ray photons ionise atoms in the surface layer of the sample by ejecting an electron from the inner electron shells of the atoms.
- Depending on the situation, the thickness of the surface layer that produces the secondary X-rays can range from a few μm (determining light elements in a sample composed mostly of heavy elements) to hundreds of μm (determining heavy elements in a sample composed mostly of light elements).
- The formed positive ions are highly excited (because of the vacancy in an inner shell) and relax very fast. Relaxation consists of two simultaneous processes: filling the vacancy with an electron from one of the next shells and emitting the released energy as a secondary X-ray photon.
- These emitted secondary X-rays are the ones that are detected by the XRF instrument and consist of photons with energies (and wavelengths) that are characteristic to the elements that are present in the sample. Every element emits X-rays with a characteristic set of photon energies and wavelengths.
Most XRF spectrometers are capable of detecting the elements of the periodic table from sodium to uranium. However, the limit of detection increases rapidly as the atomic number decreases below that of sodium.
X-ray fluorescence spectrometers of two design principles are available: wavelength dispersive X-ray fluorescence spectrometers (WD-XRF) and energy dispersive X-ray fluorescence spectrometers (ED-XRF). WD-XRF produces a spectrum by using a crystal monochromator to diffract the fluorescent X-rays, a single wavelength at a time, onto a detector that measures their intensity. ED XRF permits the fluorescence radiation emitted at all wavelengths to reach the detector simultaneously and uses a pulse-height discriminator to electronically classify the energy of the X-ray photons that strike the detector. Both designs have their advantages and limitations and are widely used.
Description of X-ray Diffraction (XRD)
The XRD method gives information about the spatial structure of crystalline materials. XRD can analyse only crystalline substances (in the case of a mixture – the crystalline components) and provides information on their structures, phases, preferred crystal orientations (texture), and other structural parameters. Generally, X-ray diffraction is based on constructive interference of monochromatic X-rays and a crystalline sample. Each crystalline substance has a unique repeating three-dimensional array of atoms and produces a unique X-ray diffraction pattern. Diffraction patterns can be used as “fingerprints” for the identification of crystalline phases.
X-ray diffraction experiments are performed by irradiating a crystalline sample with a beam of X-rays, X-rays interact with the sample in such a way that the X-rays are diffracted from the atomic planes. Because of the interference of the, rays only those that are emitted in certain directions survive (constructive interference). The remaining rays are destroyed by destructive interference.
The interaction of the incident rays with a certain set of atomic planes produces constructive interference (and a diffracted ray) when the interference angle 2Θ satisfies the following conditions, known as Bragg’s Law, which is defined as follows:
nλ=2d sin θ
where, n is an integer number (known as diffraction order), λ is the wavelength of the used radiation, and d is the distance between the atomic planes (lattice spacing).
This law relates the wavelength of electromagnetic radiation to the diffraction angle and the lattice spacing in a crystalline sample. In real crystals, there are numerous atomic planes and thus also numerous angles 2Θ that satisfy the Bragg’s law.
Each of these angles will produce a maximum (peak) on the diffraction picture (diffractogram), and for every mineral, there exists a set of characteristic peaks by which the mineral can be identified (see Fig. 1). So, both the diffraction angle and the intensity of each of the diffracted beams (diffraction peaks) are then measured, processed and counted. The intensity of diffracted X-rays is plotted as a function of diffraction angle 2θ.
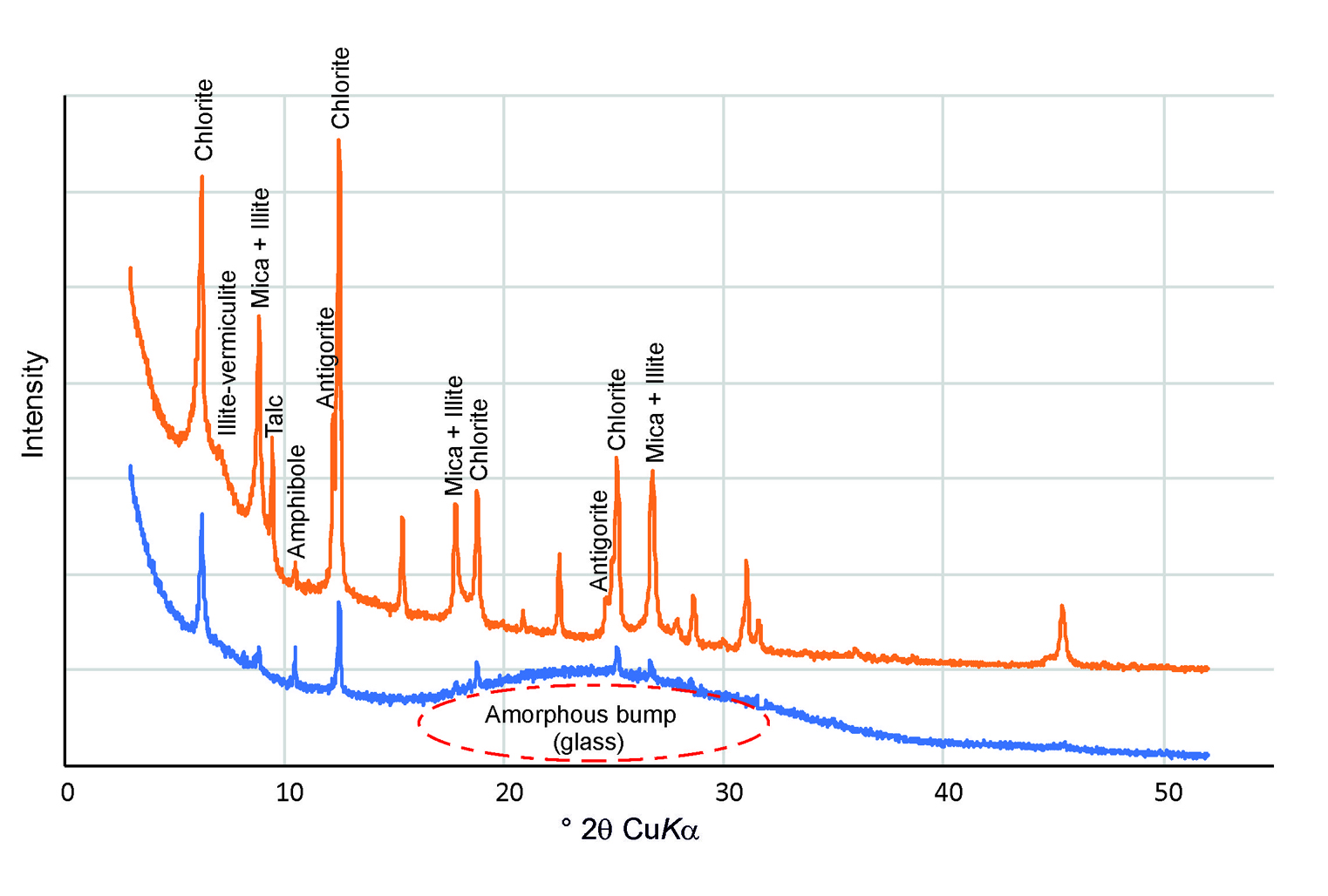
In cultural heritage research powder diffractometers are usually used. For powder diffractometer the sample is powderized in order to ensure that small crystals of all different orientations are present in the sample. For additional randomization, the sample holder is rotated. This arrangement ensures that as a result of the diffraction of a single collimated beam, all possible reflections (corresponding to all possible diffraction angles) can be observed.
The intensities of these reflections are recorded, usually with a charge-coupled device (CCD) image sensor. By scanning the sample through a range of 2θ angles, all possible reflections will be recorded due to the random orientation of the powdered material.
2. Analysis with XRD and XRF methods
In the following video, Dr Peeter Somelar introduces typical ways of sample preparation for the XRD and XRF analysis.
In the following video, Dr Peeter Somelar introduces XRD and XRF instruments and how to perform analysis using these methods.
The slides used in the video can be downloaded from here: